Nanomaterials are tiny solid particles that can be formed naturally, accidentally or in a manufacturing process. Thanks to their special and diverse properties, they can be used in a wide range of products and technologies.
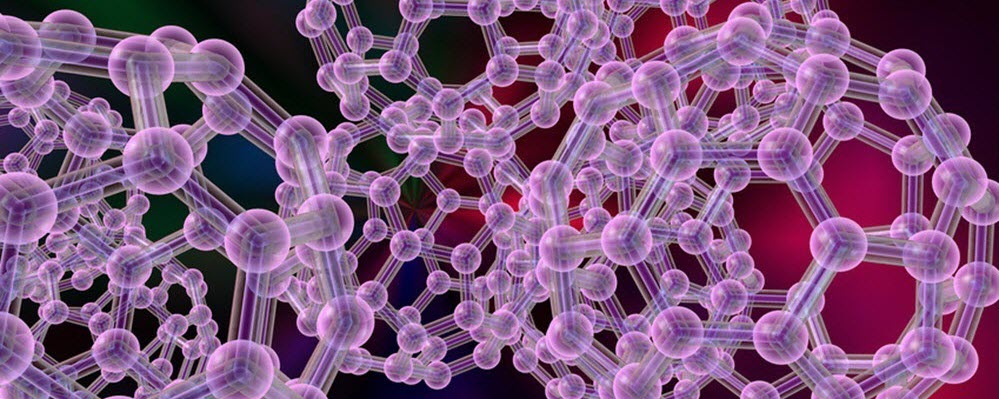
The properties of nanomaterials differ greatly from the same material in bulk form. Indeed, the behaviour and properties of nanomaterials depend not only on their composition but also on their size, shape (cylindrical, spherical, platelet, etc.), state (free particles, aggregate or agglomerate ) and surface-to-volume ratios, which are particularly high. The main properties of nanomaterials – optical, mechanical, electrical, magnetic, catalytic and hydrophobic – and their many areas of application are presented below.
Aggregate
A particle comprising strongly bonded or fused particles whose resulting outer surface is significantly smaller than the sum of the surfaces of the individual components.
Agglomerate
A collection of weakly or moderately strongly bonded particles whose resulting outer surface is similar to the sum of the surfaces of the individual components.
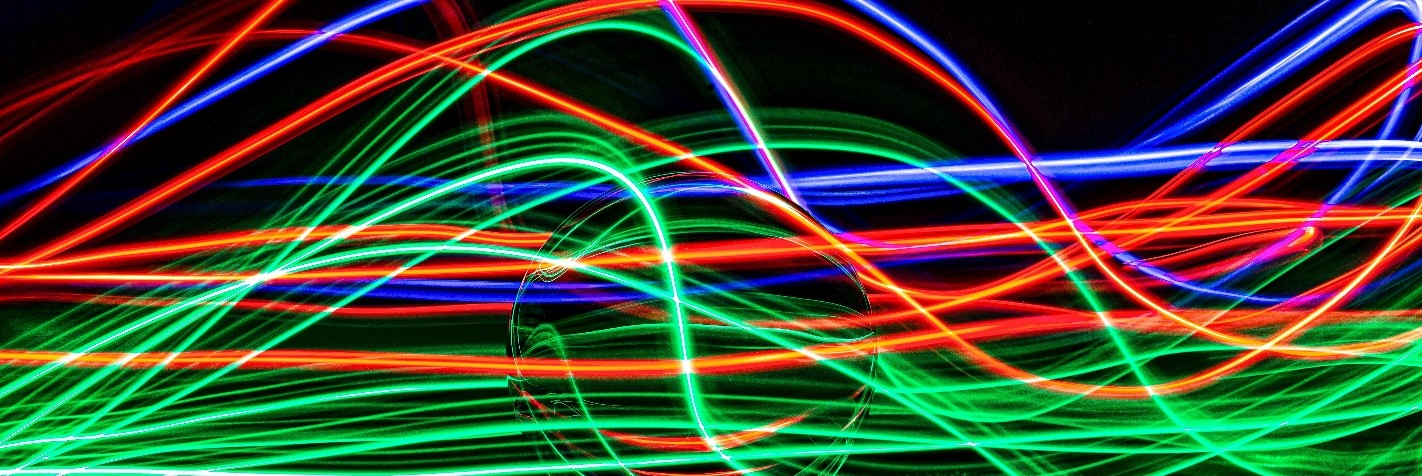
Nanomaterials have special optical properties and their interaction when exposed to light, lasers or other electromagnetic radiation (UV, etc.) may be different to that of bulk materials. The optical properties of a material are determined by processes such as absorption, transmission, reflection and emission of light. These properties can be changed by altering the shape, size or surface functionalisation of the nanoparticles, or by changing the medium in which they are found (composite material, cellular medium, etc.).
Gold nanoparticles in colloids are a good example of this. Depending on their size, gold nanoparticles appear different colours: yellow (2-5nm), red (10-20nm) or purple (>20nm). These different colours are the result of an effect known as surface plasmon resonance, which is produced by the interaction of the external electronic structure of the nanoparticle with a certain wavelength of light. This technology, known as colorimetric detection, has various medical uses. The optical properties of nanomaterials also have applications in other medical fields such as fluorescence imaging and photodynamic therapy, which is used in the treatment of certain cancers.
However, the fields of application of nanomaterials based on their optical properties are not limited to medicine. These materials are also used in processes to convert solar energy into chemical or electrical energy. In addition to their conductive and semiconducting properties, nanomaterials can save on raw materials (better efficiency per unit of surface area) and provide larger productive surfaces (solar panels) and flexible substrates.
Finally, the optical properties of nanomaterials are used in lasers, lighting (LED lamps), optical detectors and as coatings on some materials in order to filter certain types of radiation such as colour or heat.

Some nanoparticles confer valuable mechanical properties to certain materials or products, such as strength, hardness, elasticity, toughness or ductility. The mechanical properties of a material are its characteristics in different environments and under various external stresses. It is possible to use organic or inorganic nanomaterials to optimise one or more of the mechanical properties of a material.
Carbon-based organic nanomaterials include carbon nanotubes (CNTs) (single or multi-walled), nanowires, graphene, etc. These materials have considerable mechanical strength. An individual carbon nanotube has a very high Young's modulus (very high resistance to deformation) close to that of diamond. However, a wire composed of a multitude of CNTs will have a high flexibility due to Van der Waals interactions. CNTs are both very strong and highly flexible.
Examples of inorganic nanomaterials are transition metal oxides, silver or gold nanoparticles, and ferrite or silicon-based nanostructures. When certain types of zinc nanomaterial or zinc nanowire are integrated into different substrates (e.g. borosilicate glass, quartz, etc.), their mechanical strength is greatly increased. It is possible to alter the mechanical properties of materials composed of silicon-based nanostructures, such as the material's elasticity, tensile strength, rigidity or softness.
In some materials, such as concrete, the addition of silicon oxide nanoparticles (SiO2) increases strength (compression, flexion, traction) and also modifies other properties such as setting time, slump and durability. These properties are mainly determined by the effect of the nanoparticles on the chemical reactions and the degree of hydration of the cement, as well as by the formation of a more compact microstructure.
Finally, nanoparticles are also used in certain biological composite materials or in polymers. These materials are then called nanocomposites. They are used in particular to provide superior resistance to plastic fibres and films, but can also improve other non-mechanical properties (protection against UVs, fireproof materials, anti-corrosive coatings, etc.).
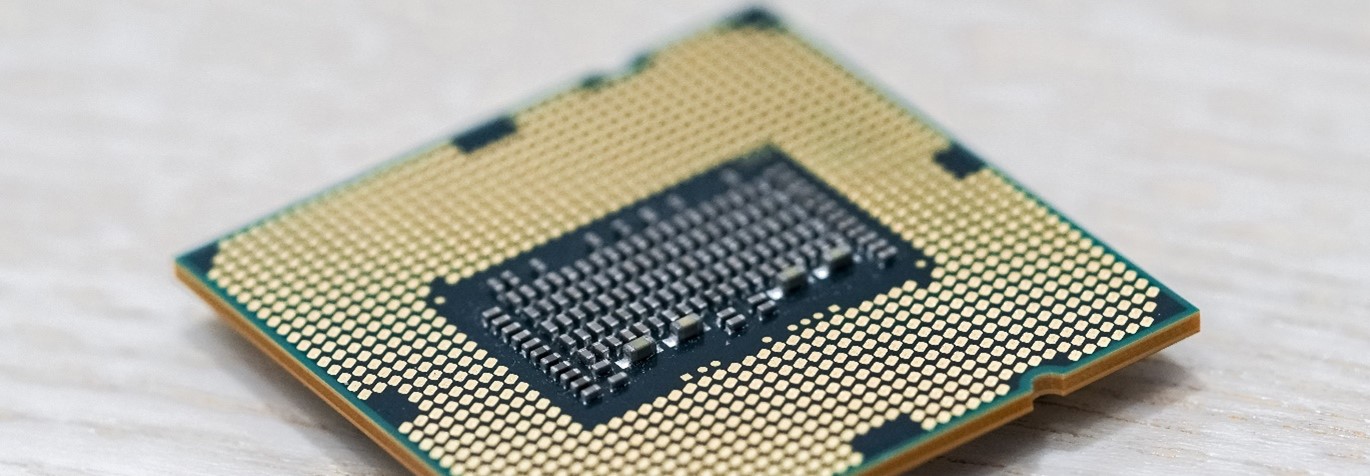
Magnetic nanomaterials are generally composed of a magnetisable element such as iron, nickel, cobalt, chromium, manganese or gadolinium. One of the particularities of nanoparticles is the superparamagnetic effect induced by their small size. In the field of nanoparticles, superparamagnetism occurs when a particle becomes magnetic under the influence of an external magnetic field but returns to a non-magnetic state when it is no longer in the magnetic field (unlike ferromagnetism). Superparamagnetism is used in a range of medical fields and in therapeutic applications, in methods to destroy cancerous cells by hyperthermia, in the administration of drugs to target specific tissue and in certain medical imaging techniques (e.g. nuclear magnetic resonance).
Nanoparticles and their magnetic properties are used in a broad range of applications, including information storage media, sample preparation in analytical chemistry (e.g. isolation and pre-concentration of analytes), fluorescence techniques (e.g. cell imaging) and the remediation of contaminated sites (e.g. as sorbents for heavy metals).
Magnetic nanoparticles also exist in nature, for example in rocks, where they can be used to determine the earth's magnetic field. Some bacteria are equipped with a nanomagnet which enables them to align themselves with the earth's magnetic field. Similarly, birds have nanoparticles in their beaks that are sensitive to the Earth's magnetic field, enabling them to navigate.

The electrical properties of a material are characterised by its conductivity (defined as the amount of electrical charge transferred per unit time) and its electrical resistivity (the opposite of conductivity). Materials are classified as metallic, semiconducting or insulating according to their degree of electrical conductivity/resistivity.
The conductivity of CNTs depends on the way their individual hexagon units are arranged. When these are in an ‘armchair’ configuration, they conduct electric current even more readily than copper. When CNTs are made up of hexagons linked in a zig-zag or chiral configuration, they behave like semi-conductors. Semi-conductors have a range of applications, including as transistors in electronic devices such as computers and gas detectors. CNTs’ conductive properties result from the electron delocalisation made possible by the carbon double bonds (pi bonds). They can be modified by binding other molecules to these nanotubes.
Metal-based nanomaterials such as gold, silver, certain cobalt oxides and other alloys and composites have very interesting properties not only with regard to electrical but also thermal conductivity. This area of research is important for the conversion of thermal energy into electrical energy and vice versa, for example for energy production and refrigeration purposes. Research groups are working on nanomaterials and composites containing nanomaterials based on silicon, bismuth telluride, binary skutterudite and silver. Certain so-called ‘thermoelectric’ materials could, for example, improve electricity production and energy recovery during waste incineration.
Nanomaterials could also improve electricity storage capabilities by improving various battery properties. Indeed, the use of certain nanomaterials could improve lithium batteries by increasing their power density (power versus mass), reducing the risk of fire or increasing the speed of recharging/discharging. These nanomaterials could for example increase the efficiency of electric or hybrid vehicles.

Nanomaterials have different properties that can increase the speed, yield or stereoselectivity of a chemical reaction. This field of research and industrial application is called nanocatalysis. The main catalytic properties of nanomaterials come from their surface and quantum effects. The surface effects are due to the fact that the specific surface area per unit volume of nanomaterials is much higher. This property allows the nanocatalyst to interact more with the surrounding molecules than a conventional catalyst. The increased reactivity of nanocatalysts can also be explained by quantum effects induced by their size. This phenomenon results from the confinement of electrons in particles of smaller dimensions than their bulk counterpart. As the particle size decreases, the specific surface area increases and a quantum effect occurs, leading to profound changes in the properties of the materials.
These nanocatalysts are used in many areas of the petroleum and pharmaceutical industries and can be easily separated and recycled. A telling example is gold. This precious metal is almost inert in its macroscopic form. However, in its nanometric form, it becomes a very effective catalyst in the fight against air pollution and for air quality controls (air purification, monitoring probes), in hydrogen-powered fuel cells or for the synthesis of food additives such as gluconic acid.
Finally, some nanoparticles have photocatalytic properties that can be used to clean up the environment of pollutants or hazardous waste. These processes are made possible by the use of semiconductors composed of metal oxides such as titanium dioxide (TiO2). These nanomaterials facilitate the mineralisation (oxidation) of certain pollutants such as chlorinated aliphatic or aromatic hydrocarbons into other molecules that are less or not at all toxic to the environment.

The hydrophobicity or hydrophilicity of a natural or artificial material is defined by the angle that a drop of water forms on its surface. This angle defines whether or not the material will get wet when in contact with water. When the angle formed by a drop of water on a material is greater than 90°, the material is not wet and is defined as hydrophobic. When the angle formed between the drop of water and the material is less than 90°, the water spreads over the surface and this is characterised as hydrophilic.
Some natural or artificial nanomaterials have superhydrophobic qualities. This means that a drop of water forms an angle greater than 150°, allowing it to run off very easily from a surface made of such nanomaterials. Superhydrophobic materials are studied and used on building surfaces for their anti-fouling and self-cleaning properties, in gas pipelines to protect against corrosion, or in microfluidic technical devices (e.g. for medical systems).
Last modification 16.01.2023
Contact
Federal Office of Public Health FOPH
Chemical Products Division
Schwarzenburgstrasse 157
3003
Bern
Switzerland
Tel.
+41 58 462 96 40